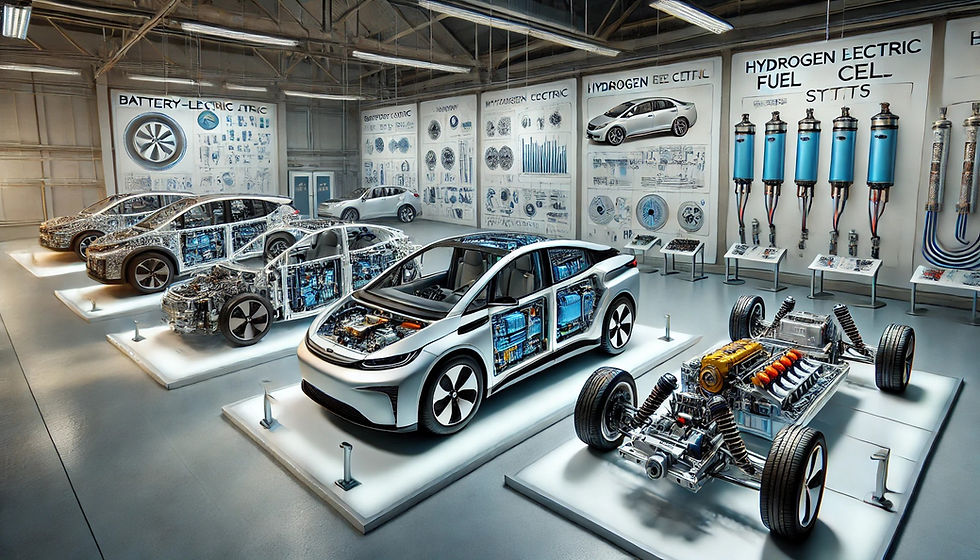
Electromobility is experiencing an unprecedented upswing. Governments around the world are promoting the transition to sustainable powertrains, and car manufacturers are investing billions in new technologies. While the public is increasingly convinced that the future will be electric, the question is whether the masses really understand the differences between the different types of electric powertrains. After all, not all electrification is the same – the range of technologies is enormous and offers solutions for a wide variety of requirements. This article provides a comprehensive overview of the different electric actuators, how they work, advantages and disadvantages, and their potential applications.
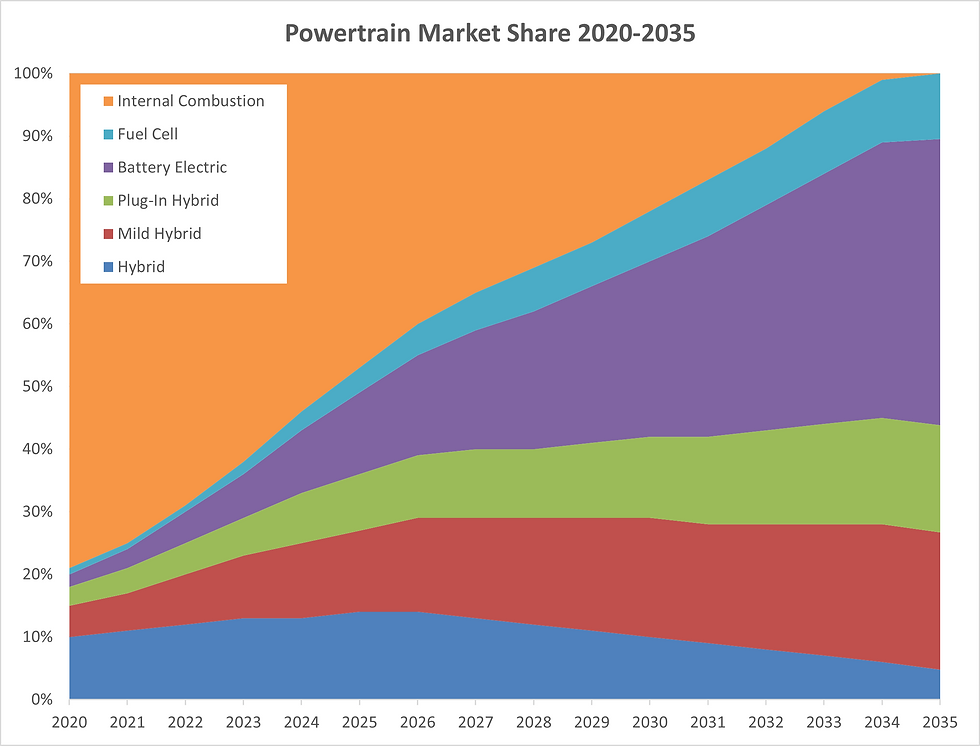
Hybrid drives: The entry into electrification
Hybrid drives combine a combustion engine with an electric motor, which is primarily used to support the combustion engine when starting off and accelerating. The battery is charged while driving through brake energy recovery. Vehicles such as the Toyota Prius or the Honda Insight are considered pioneers of this technology. Hybrid cars offer significant fuel savings and reduced CO₂ emissions. However, their purely electric range is very limited, and they are often heavier than pure combustion engines. They are ideal especially for city traffic and short distances, where frequent stop-and-go occurs.
Mild hybrids: electrification on a small scale
Mild hybrid systems are a simplified form of hybrid drives. Here, a small electric motor takes over supporting functions, such as starting the combustion engine or assisting with acceleration. However, purely electric operation is not possible. The Audi A6 and the Mercedes-Benz C-Class are examples of vehicles with mild hybrid technology. These systems offer an improvement in efficiency at comparatively low additional costs, but are not suitable for emission-free driving.
Plug-in hybrids: The bridge between combustion engine and electric
Plug-in hybrids, such as the BMW 330e or the Volvo XC60 Recharge, combine a combustion engine and electric motor with the possibility of charging the battery via the power grid. They offer an electric range of 30 to 60 kilometers, making them ideal for city traffic. However, they require charging infrastructure and disciplined use to take full advantage of their benefits. High costs and the additional weight of both drives are further challenges.
E-axles: Compact efficiency
The e-axle integrates the electric motor, transmission and power electronics in a compact unit. This technology is often used in SUVs and electric vehicles such as the Audi e-tron or the Mercedes EQC . It offers space-saving, high power density and flexibility in vehicle design. Nevertheless, the complex design requires higher development investments.
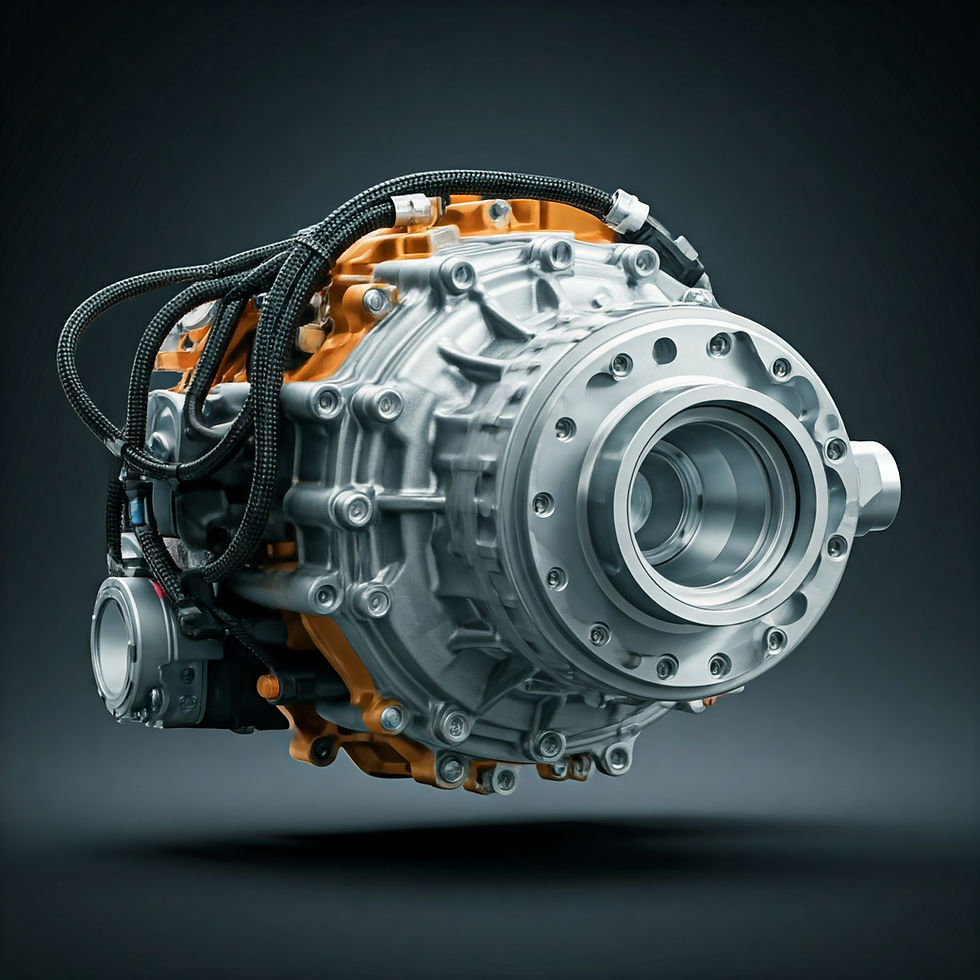
Serial hybrids: The combustion engine as a power generator
In serial hybrids, such as those found in the Chevrolet Volt, the combustion engine does not drive the wheels directly, but generates energy for the electric motor. This enables optimal efficiency of the combustion engine while driving remains purely electric. However, there are energy losses due to the conversion, which can reduce efficiency compared to other systems.
Parallel hybrids: The power of two engines
Parallel hybrids work with a combustion engine and an electric motor, both of which can drive the wheels directly. This allows for flexible power delivery and better acceleration. Examples of vehicles with parallel hybrid drive are the Hyundai Ioniq Hybrid and the Kia Niro Hybrid. This technology is ideal for dynamic driving situations, but technically more demanding and heavier.
Range extenders: More flexibility for electric vehicles
A range extender is a small combustion engine that is used in vehicles such as the BMW i3 REx to charge the battery and increase the range. This solution minimizes range anxiety while still enabling largely electric driving. However, the extra weight is a disadvantage, and locally emission-free driving is not possible when the combustion engine is activated.
Fuel cell vehicles: Hydrogen as the key
Fuel cell vehicles such as the Toyota Mirai or the Hyundai Nexo generate electrical energy through the chemical reaction of hydrogen and oxygen. These vehicles are emission-free and offer a long range and short refuelling times. However, the expensive hydrogen infrastructure and the limited availability of filling stations still pose significant hurdles.
Wheel hub motors: drive directly on the wheel
Wheel hub motors, such as those used in concept vehicles such as the Riversimple Rasa , integrate electric motors directly into the wheels. This saves space and maximizes efficiency. However, the unsprung mass increases the demands on the chassis, and the control is more complex.
E-Traction and E-Transmission: The Future of Drive Architecture
E-transmissions optimize the performance of electric motors and offer variable power delivery. Vehicles such as the Porsche Taycan benefit from this technology, which is mainly used in high-performance and commercial vehicles. Despite the efficiency gains, the costs and development effort are high.
Comparison Table of Drive Types
Drive type | Advantages | Disadvantages |
Hybrid | Fuel-saving, no charging infrastructure required | Limited electric range, heavier than combustion engines |
Mild-Hybrid | Cost-effective, increased efficiency | No purely electric operation possible |
Plug-In-Hybrid | High flexibility, locally emission-free driving possible | High costs, charging infrastructure required |
E-axles | Space saving, high power density | Complex development, higher costs |
Serial Hybrids | Optimum efficiency, all-electric driving possible | Energy losses due to conversion |
Parallel Hybrid | Flexible power delivery, dynamic driving | Higher weight, complex design |
Range Extender | Minimized range anxiety, mostly electric | Higher weight, not locally emission-free |
Fuel cells | Emission-free, long range | Expensive infrastructure, limited petrol station availability |
The Radnaben engine | Maximum efficiency, space saving | Higher unsprung mass, complex control |
E-transmission/e-traction | Increased efficiency, high variability | High development costs, cost-intensive |
Pure electric vehicles: architectures, drive concepts and their significance for the future
Electromobility is considered the key technology for a sustainable transport transition. Pure electric vehicles, also known as battery electric vehicles (BEVs), are at the heart of this development. In contrast to hybrid drives, they completely dispense with a combustion engine and rely exclusively on electrical energy as the driving force. These vehicles symbolize an emission-free future and offer innovative technical solutions that are revolutionizing not only the automotive industry, but also the way people experience mobility. The diversity of architectures, drive concepts and battery technologies shows how different the requirements and possible applications for electric vehicles can be. From compact city runabouts to high-performance long-distance models, each concept has its own approach to making mobility more efficient and sustainable. Their diversity ranges from different battery sizes and drive concepts to innovative vehicle architectures that have been specially developed for electric operation. In the following, the different variants and architectures of pure electric vehicles are presented in detail
Electric Vehicle Architectures
The architecture of an electric vehicle is fundamentally different from that of a vehicle with an internal combustion engine. The elimination of classic drive components such as the engine compartment, transmission or exhaust systems gives designers and engineers more freedom in the development of new vehicle concepts.
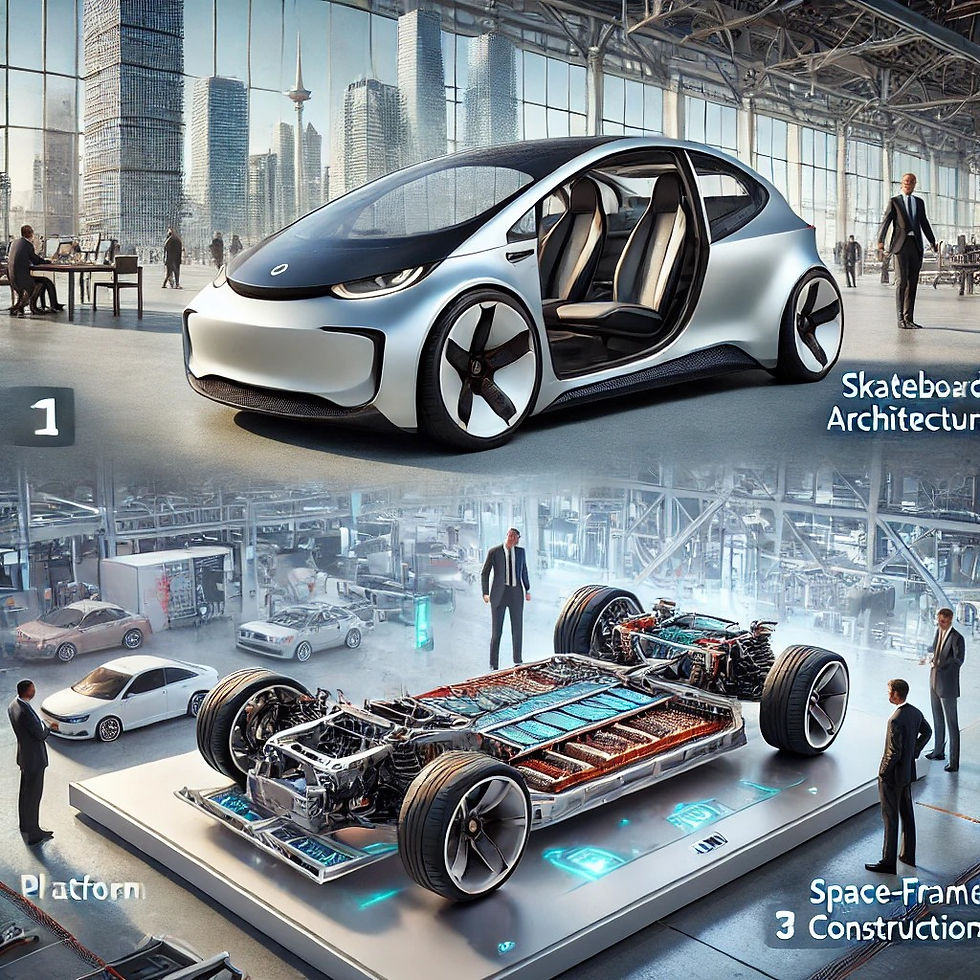
Skateboard architecture One of the most famous architectures is the so-called skateboard architecture. In this approach, the battery is integrated flat into the vehicle floor, while the electric motors are positioned directly on the axles or in the wheels. This architecture, used by manufacturers such as Tesla in models such as the Model 3 or Rivian in their R1T pick-up model, offers numerous advantages. The low-lying battery ensures a low centre of gravity, which improves driving dynamics, and enables optimum use of interior space. In addition, this design offers a high degree of modularity, so it can be adapted for different vehicle types and sizes. However, the technical requirements, especially the cooling of the battery and its integration into the vehicle structure, are a challenge that requires high investments.
Platform integration Another approach to the development of electric vehicles is platform integration, such as that pursued by Volkswagen with the Modular Electric Drive Matrix (MEB). Vehicles such as the Volkswagen ID.4 are based on this platform, which allows for standardized positioning of batteries and motors. This concept aims to reduce development costs by leveraging economies of scale. At the same time, the platform remains flexible enough to build different vehicle models on it. However, the downside of this approach is the limited design freedom compared to architectures that are developed specifically for a single model.
Space frame constructions Another innovative concept is the space frame construction, which uses lightweight materials such as aluminum or carbon to compensate for the additional weight of the battery. Vehicles such as the BMW i3 use this design, which leads to higher energy efficiency due to lower weight. However, the production costs for such materials and manufacturing processes are significantly higher, which has so far limited their use to premium vehicles.
Different drive concepts
The choice of drive concept plays a decisive role in the performance and efficiency of an electric vehicle.
Single motor with front-wheel drive Compact models such as the Nissan Leaf or the Renault Zoe often use a single electric motor with front-wheel drive. This concept is simple and inexpensive, but offers limited traction and a less sporty ride. Single motor with rear-wheel drive In contrast, vehicles such as the Tesla Model 3 Standard Range rely on rear-wheel drive, which allows for better weight distribution and a more dynamic driving experience by positioning the engine on the rear axle.
Dual motor with all-wheel drive For even higher performance, many premium vehicles use a dual motor with all-wheel drive. Models such as the Audi e-tron or the Jaguar I-PACE use this concept, in which each engine drives an axle. This allows precise control of the power transmission to each wheel, which is particularly advantageous in demanding road conditions or sporty riding. However, the additional technology and control systems increase production costs.
Wheel hub motors, as tested in some concept vehicles, drive the wheels directly and eliminate mechanical losses from transmissions and axles. Vehicles like the Lightyear 0 use this technology. Wheel hub drives have the advantage of being very efficient and space-saving. However, there is the problem of unsprung masses and a much more sophisticated control.
Battery technologies and ranges
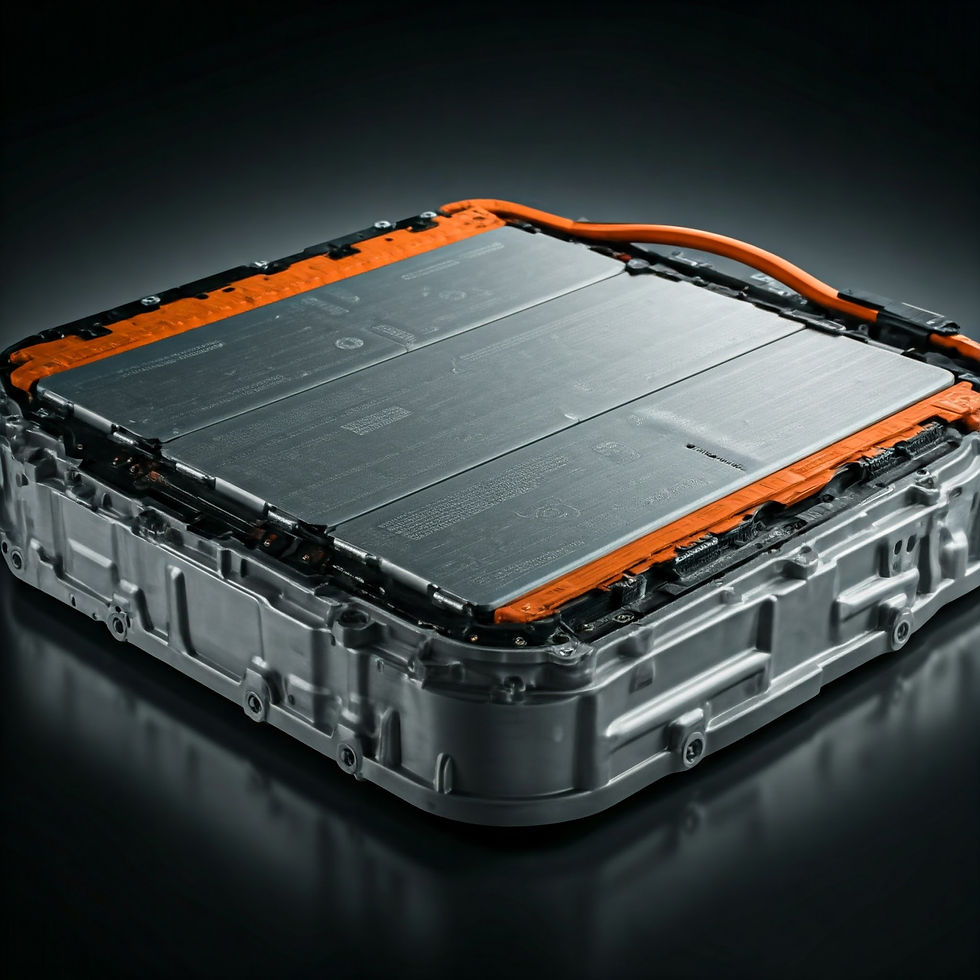
In addition to drive technology, the battery is the central element of an electric vehicle. The battery is the heart of every electric vehicle. Their capacity, chemical composition and management system determine range and charging speed.
Lithium-ion batteries Most current models use lithium-ion batteries, which are characterized by high energy density and a long service life. Vehicles such as the Hyundai Kona Electric or the Kia EV6 successfully use this technology. However, one disadvantage of these batteries is the limited availability of raw materials such as lithium and cobalt, as well as the challenges of recycling.
Trend: Lithium-ion batteries are currently the dominant technology with the largest market share. It will remain dominant until 2030, but the share could slowly decline as other technologies gain traction. Continuous improvements in energy density, service life and safety are expected. 2030-2050: Probably still a significant share, but no longer the dominant technology. Specialized applications could continue to rely on Li-Ion.
Solid-state batteries: A promising alternative is solid-state batteries, which are currently under development. These batteries promise even higher energy density, shorter charging times and improved safety. Manufacturers such as Toyota are working on the introduction of this technology, but it is still associated with high production costs.
Trend: Currently mainly in the R&D stage, very low market share. By 2030: Expected market entry and slow growth. Challenges in mass production and cost reduction must be overcome. 2030-2050: Significant growth and potentially significant market share if the technological and economic hurdles are overcome. Could play an important role in premium electric vehicles and other demanding applications.
LFP (Lithium Iron Phosphate) batteries Another variant is lithium iron phosphate (LFP) batteries, which are mainly used in low-cost electric vehicles such as the Tesla Model 3 Standard Range Plus. They offer high thermal stability and a long service life, but have a lower energy density compared to conventional lithium-ion batteries.
Other technologies (e.g. lithium-sulfur, sodium-ion): These technologies are still in an early stage of development, but have potentially high energy densities and/or advantages in terms of raw material availability. It is difficult to predict when and to what extent they will affect the market.
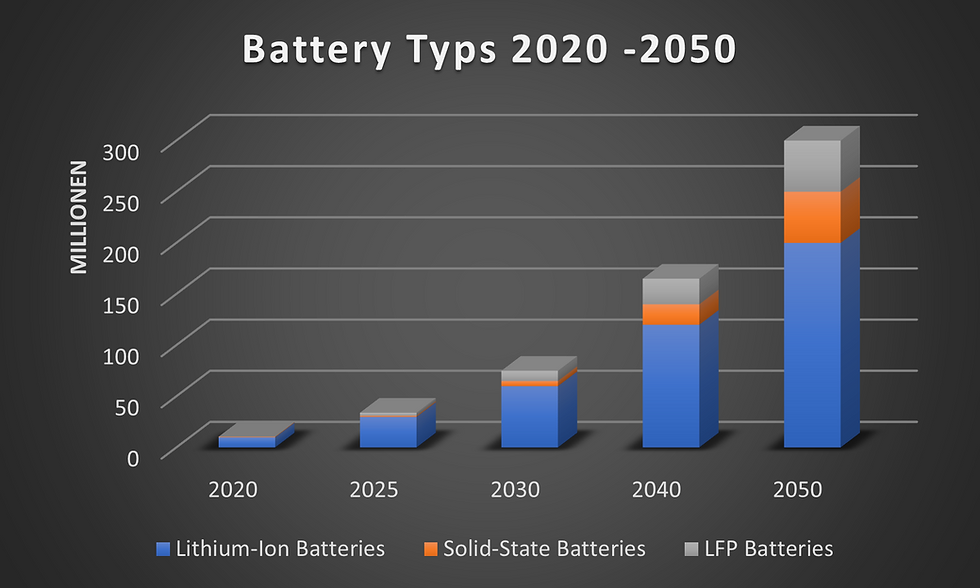
Pure electric vehicles such as the Tesla Model S Plaid, the Volkswagen ID.3 or the Porsche Taycan show how different the approaches and target groups in this segment can be. While Tesla is focusing on maximum performance and range with the Model S, Volkswagen is focusing on affordability and the mass market with the ID.3. The Porsche Taycan, on the other hand, combines electric mobility with sporty dynamics and luxury.
The diversity of architectures and drive concepts makes it clear that electric vehicles are more than just a replacement for combustion vehicles. They open up new possibilities in vehicle design and create a sustainable basis for the mobility of the future. With the advancement of battery technologies and the increasing availability of charging infrastructures, the transition to electric mobility is becoming increasingly attractive and accessible. Pure electric vehicles are a decisive step on the way to an emission-free transport system that will change mobility in the long term.
Conclusion and outlook
The electrification of the automotive sector is revolutionizing mobility, but it is also creating a complex technological fabric. From hybrids to fuel cells to all-electric vehicles, each type of drive offers specific advantages and disadvantages. While plug-in hybrids are currently a bridging technology, fuel cells and new concepts such as wheel hub motors and e-axles could dominate the mobility of the future. In the long term, a mixture of different technologies can be expected, which will be used depending on the application. One thing is clear: electric drives will not only change vehicles, but also our understanding of mobility and its impact on the environment.
Comments